Mikrofluidik
Helix Formation of Semiflexible Polymers in Flow through Structured Microchannels
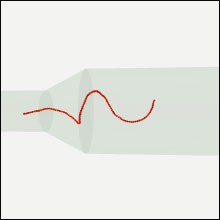
Buckling is a common phenomenon in slender bodies, such as long filaments and thin sheets, under an external load. Typically, buckling transitions are considered under equilibrium conditions. The transport of semiflexible polymers in microchannels and capillaries presents a new opportunity to study the non-equilibrium behaviour of such filaments. Vice versa, a detailed understanding of the dynamical processes involved in this type of transport is of paramount importance in many applications, in particular for the flow behaviour of many biologically-relevant polymers, such as DNA, actin filaments, and microtubules. Non-equilibrium instabilities can appear under various conditions. We investigate flow fields of spatially varying flow strength. Such a situation is easily realized in flows through spatially-structured microchannels. In mesoscale hydrodynamic simulations, we observe the buckling of a semiflexible polymer as it enters a wider channel section. Buckling is often the first step in the formation of more complex structures. Indeed, we observe a subsequent flow-induced helical coiling of the polymer. The helix properties are studied in terms of their dependence on the diameter ratio of the channel, the polymer bending rigidity, and the flow strength.
Rotating Colloids in Microchannels
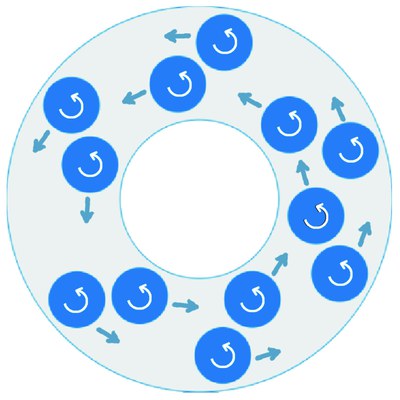
Externally actuated and self-propelled micro- and nano-rotators show an intriguing variety of non-equilibrium structure formation and dynamics. Examples of such systems include super-paramagnetic colloidal particles in a rotating magnetic field, dipolar colloids in a rotating electric field, colloidal dimers rotated by laser tweezers, and biological swimmers such as Volvox algae. The collective behavior of rotator suspensions is governed by hydrodynamic interactions.
We employ mesoscale hydrodynamics simulations to address the fundamental question how the rotation of the colloids about their own axes can be converted into a translational motion by symmetry breaking in linear and ring channels. In straight channels, we observe a two-way traffic, but -- for symmetry reasons -- no net transport. However, by keeping some colloids fixed near one of the two walls, net transport can be achieved, allowing the control and switchability of the flow in complex micro-channel networks. For ring channels, the channel curvature generates an overall translational velocity, which depends on the channel geometry.
Vesicles in Structured Microchannel Flows
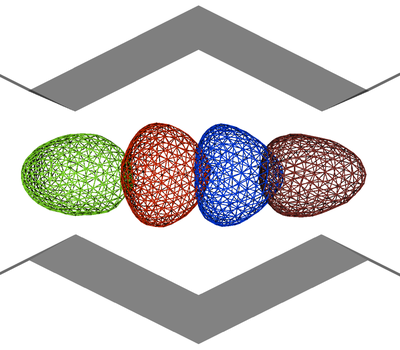
The recent development of microfluidic techniques allows the investigation and manipulation of individual cells and vesicles. There are many potential applications such as blood diagnosis on chip. Under steady flows in homogeneous microchannels, vesicles and RBCs deform into a bullet or parachute shape. Compared to these steady-flow conditions, the vesicle dynamics in time-dependent flow is much less explored.
We study the dynamics of fluid vesicles under flow in microchannels, in which the width varies periodically along the channel. Three types of flow instabilities of prolate vesicles are found. For small quasi-spherical vesicles -- compared to the average channel width -- we predicts a transition from a state with orientational oscillations of a fixed prolate shape to a state with shape oscillations of symmetrical ellipsoidal or bullet-like shapes with increasing flow velocity. For larger non-spherical vesicles, we find new shapes with two symmetric or a single asymmetric tail.
Red Blood Cells in Capillary Flow
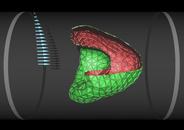
The flow properties of blood are determined to a large extent by the presence of red blood cells, which constitute about 50% of its volume. In diseases such as diabetes mellitus, red blood cells have a reduced deformability, which enhances the flow resistance of blood. We study the deformation of red blood cells by a simulation technique, which combines a particle-based mesoscopic hydrodynamics method with a dynamically-triangulated membrane model (with shear and bending elasticity). As flow velocity increases, model red blood cells in microcirculation are found to transit from a non-axisymmetric discocyte to an axisymmetric parachute shape (coaxial with the flow axis), as shown in the figure. In contrast, a fluid vesicle (without shear elasticity) is found to transit from a discocyte to a cigar-shaped prolate ellipsoid. Both shape transitions reduce the flow resistance. Our results are in good agreement with experiments on red blood cells.