Phenomena
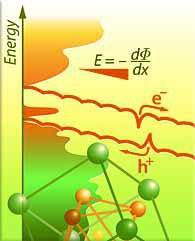
Our research is focussed on electronic phenomena in electronic oxides, in particular transition metal oxides, and dedicated electronically active organic molecules. The phenomena include electronic and ionic transport, electron transfer reactions, electrochemical redox processes, ferroelectricity and piezoelectricity, electron correlation effects and magnetic phenomena, as well as space charge formation and field effects. Most of our projects aim at progress in the fundamental understanding of these phenomena on the nano-scale and at the discovery and the exploration of novel electronic functions.
nanoscale electron transfer - towards redox-based terabit memories
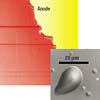
In a range of oxides, for example transition metal oxides, field-induced ion migration takes place at relatively low temperatures in extended defects within the crystal lattice or in amorphous systems. This ion migration is typically accompanied by redox reactions at the electrode interface and electron transfer reactions. In the case of the migration of oxygen ions, the redox reaction occurs in the transition metal cation sublattice and often leads to insulator-metal transitions. The formation and dissolution of electron conducting channels may possibly be used for non-volatile resistive memories with integration densities up to Terabits per sqcm. Our research aims at the elucidation of the molecular structure of fast ion migration paths, the formation of space charge regions, details of the redox reaction in the oxide, and a microscopic view of the charge transfer reaction at the electrode interface. This research field is highly interdisciplinary involving solid-state and surface physics, electronic engineering as well as nanotechnology.
polar oxides - exploiting ferroelectric and piezoelectric effects
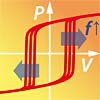
Ferroelectricity is a cooperative phenomenon in the crystal lattice of polar materials, e. g. specific perovskite structured oxides ABO3 where, for example, A = Ba, Pb, etc. and B = Ti, Zr, etc. Ferrolectric.polarization arises from a tiny, spontaneous displacement of the cation and the oxygen ion sub lattices, cooperative for all lattice cells within a ferroelectric domain. Our research aims at finding and understanding the scaling limits at which the ferroelectric effect fades due to a shortage of interacting unit cells. In addition, we are studying the effect of the atomic structure and composition of the electrode interface on the local polarization, the formation of the depolarizing field, and multiferroic interactions. Furthermore, we are interested in the dynamics of the ferroelectric switching, i. e. in the details of the domain wall motion and the kinetics of the polarization reversal. As a basic concept of our projects, our goal is to use the progress in the physics of ferroelectrics to understand and improve the performance of devices such as ferroelectric random access memories or piezoelectric actuators.
complex oxide interfaces - valency changes and metal-insulator transitions

Transition metal oxides (TMO), in particular perovskite-structured crystals, exhibit a broad variety of interesting phenomena such as charge- and spin-density waves, Jahn-Teller distortions, colossal magnetoresistance, unusual metal-insulator transitions, and superconductivity. These phenomena arise from the d-orbitals of TM cations which are both very tightly localized and highly directional, leading to narrow electronic bands and strong electron-electron interaction. In recent years, more unexpected effects have been discovered in layered structures, hetero-interfaces, and artifical superlattices which comprise 2-D electron gases, charge ordering, electronic and atomic reconstructions, correlated electron effects, as well as field effects. We aim at applying our expertise in the area of lattice disorder thermodynamics of transition metal oxides to oxide heterostructures and artifical superlattices in order to explore the physics of the 2-D effects and related phenomenon. In addition, we are interested in the interplay between ferroelectric polarization and hetero-interface charges. Despite the fact that this research is curiosity-driven and fundamental, we are searching for paths on which the results of these studies can be utilized for novel nanoelectronic devices.
electrochemistry at the nano scale
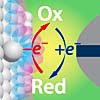
In electrochemical processes, the size reduction of structures from the micrometer to the nanometer scale leads not only to a miniaturization of fabricated or modified units, but also has tremendous fundamental implications. Processes at the nano scale typically take place below dimension of the space charge regions in solid electrolytes or the diffusive double layer in liquid electrolytes, so that the requirement of an overall charge neutrality can be dropped and new degrees of freedom are opened. We are interested in electrochemical metal deposition and metal dissolution reactions at the scale of few nanometers for non-volatile resistive switching memories which show the potential of intergration densities up to the Terabits per sqcm range - similar as the oxide based redox systems. In addition, we use electrochemical processes at the fabricate nano gap for complex molecules which are interesting because of their electronic functions. Furthermore, we pursue a research activity in the area of micro Li ion batteries.
molecular electronics - concepts beyond the CMOS world
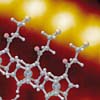
Specific organic molecules are capable of conduction and of storing single (or few) electrons which, in principle, can be controlled and manipulated. Molecules can be tailor-made by chemical synthesis in order to tune their electronic properties and to create nanometer-sized objects with dedicated electronic functionalities. In addition, they have the potential to self-organize on surfaces to regular 2-D patterns as well as to well-defined 3-D supramolecular objects. In fact, because of their tiny size to the order of one nanometer, they appear to be the ideal building blocks for designing future electronic devices with integration densities orders of magnitude beyond the CMOS concept of today. However, many fundamental questions have to be answered along the road of this evolution such as an unequivocal assignment of electronic functions to the molecular structure, controlled electronic contacts, molecular interconnects, etc. We are particularly interested in exploring the electron transport and storage properties of individual molecules. We investigate charge-controlled conformation changes, electronic interaction between metal nano-clusters and attached molecules, and excited states of cluster-molecule complexes for potential use as intramolecular logic gates. In cooperation with theory groups we achieve a complete quantum physical picture of the electronic behaviour of our molecular objects.