Blood Flow
Drug Delivery by Micro- and Nanoparticles in Blood Flow
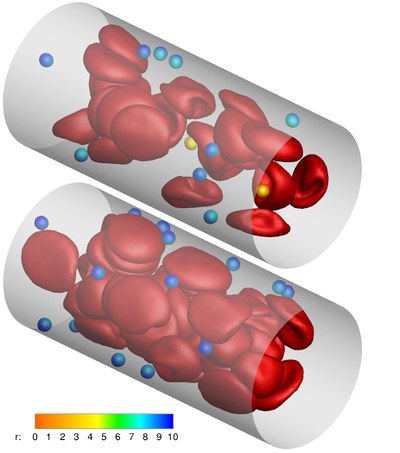
A promising strategy for early detection and efficient treatment of diseases, such as cancer, is the use of targeted carriers. After injection into the blood, ideal carriers would migrate to the wall, in a process called “margination”, in order to be able to scan the blood vessel for indications of the disease.
After recognizing the affected tissue, the carriers would adhere to the wall, and imaging agents and/or drugs would be released and transported into the tissue.We investigate the carrier margination in blood flow depending on particle size and shape, and the flow parameters like flow rate and hematocrit (volume fraction of red blood cells). We employ a mesoscopic particle-based hydrodynamics simulation technique, combined with a triangulated-network model for red blood cells (RBCs) and carriers. Due to a lift force — the hydrodynamic interaction of particles with the vessel wall — the deformable RBCs migrate away from the wall and a RBC-free layer close to the wall develops, into which the less deformable carriers can marginate. Margination is found to improve with decreasing channel size, increasing hematocrit, and with increasing shear rate.
Furthermore, micron-sized particles are preferable over nano-sized particles, and ellipsoids are more efficient than spheres. These predictions are supported by recent experiments on particle margination and adhesion.
Blood Rheology
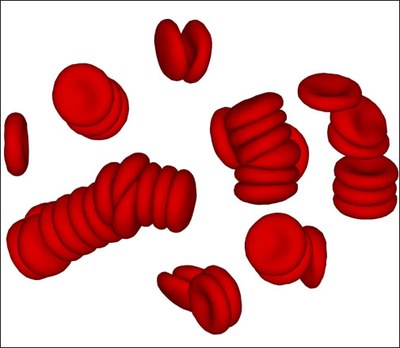
Rheological and material properties of blood directly affect blood flow characteristics, which are of importance in many bio-medical and engineering applications.
Blood consists of red blood cells (RBCs), predominant by volume, and a small fraction of other cells and proteins suspended in the plasma. Blood rheological properties are governed by the cell mechanical properties, hydrodynamic interactions as well as by RBC aggregation.
We study blood rheology using a particle-based mesoscopic simulation technique. The blood model accurately predicts the dependence of blood viscosity on shear rate and hematocrit. Specifically, RBC aggregation interactions lead to the formation of reversible rouleaux structures and to a tremendous increase of blood viscosity at low shear rates.
The simulations provide the first quantitative estimate of the magnitude of adhesive forces between RBCs and to relate the non-Newtonian blood behavior with the suspension's microstructure, deformation and dynamics of single RBCs. The model can easily be generalized to study a much wider class of complex fluids including capsule and vesicle suspensions.
Clustering and Arrangement of Red Blood Cells in Microcapillaries
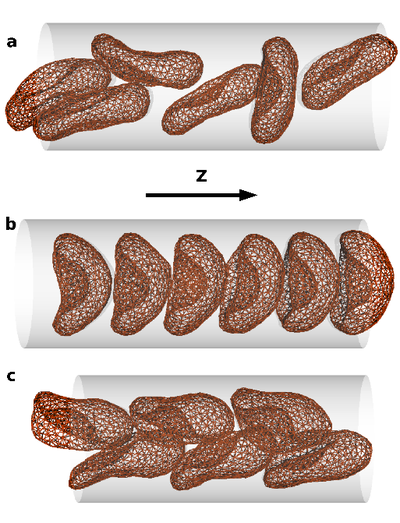
The collective behavior of several red blood cells (RBCs) in narrow capillaries determines their flow-induced morphology, arrangement, and effective viscosity. Of fundamental interest here is the relation between the flow behavior and the elasticity and deformability of RBCs, their long-range hydrodynamic interactions in microchannels, and thermal membrane undulations.
We study these mechanisms in an in silico model, which combines a particle-based mesoscale simulation technique for the fluid hydrodynamics with a triangulated-membrane model. The essential control parameters are the volume fraction of RBCs (the tube hematocrit), and the flow velocity. At very low hematocrit, the deformability of RBCs implies a flow-induced cluster formation above a threshold flow velocity. At higher hematocrits, three phases are predicted: disordered biconcave-disk-shaped RBCs, parachute-shaped RBCs aligned in a single file, and slipper-shaped RBCs arranged in parallel interdigitated rows. This is relevant for potential applications, such as blood diagnosis and cell sorting in microfluidic devices.
Red blood cell in capillary flow
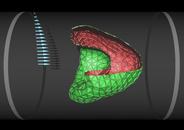
The flow properties of blood are determined to a large extend by the presence of red blood cells, which constitute about 50% of its volume. In diseases such as diabetes mellitus, red blood cells have a reduced deformability, which enhances the flow resistance of blood. We study the deformation of red blood cells by a simulation technique, which combines a particle-based mesoscopic hydrodynamics method with a dynamically-triangulated membrane model (with shear and bending elasticity). As flow velocity increases, model red blood cells in microcirculation are found to transit from a non-axisymmetric discocyte to an axisymmetric parachute shape (coaxial with the flow axis), as shown in the figure. In contrast, a fluid vesicle (without shear elasticity) is found to transit from a discocyte to a cigar-shaped prolate ellipsoid. Both shape transitions reduce the flow resistance. Our results are in good agreement with experiments on red blood cells.