Microswimmers
Sperm Motion and Navigation in Structured Microchannels
Sperm cells swim in a fluid by the wave-like beating of their flagellum. In narrow channels – as in the female reproduction tract, or in microfluidic devices for the selection of viable sperm for in vitro fertilization – the directed motion is strongly affected by the interaction with the channel walls and the flagellum-induced fluid flows.
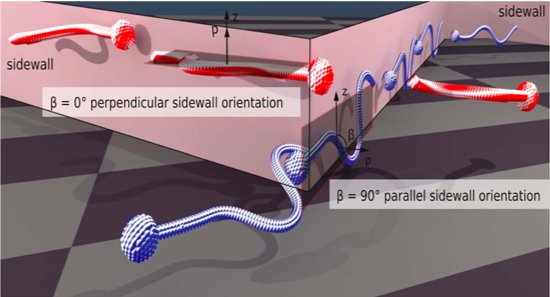
Computer simulations (which employ a mesoscale hydrodynamics approach for the fluid flow) show that in structured – curved or zigzag-shaped – microchannels, the accumulation of sperm at the wall depends strongly on the modulation of the channel geometry and the beat shape of flagellum. In particular, the orientation of the beat plane relative to the side wall plays an important role, where detachment is favored for parallel orientation. Therefore, the accumulation at curved side walls is facilitated by three-dimensional (i.e. non-planar) beat patterns. Sperm can follow weakly curved walls, but detach at sharp corners.
Altogether, the simulation results show that passive guidance and sorting of sperm in microchannels is dominated by the steric interaction with the walls, whereas hydrodynamic interactions play a minor role.
Reference: S. Rode, J. Elgeti, and G. Gompper, Sperm motility in modulated microchannels. New Journal of Physics 21, 013016 (2019), DOI: 10.1088/1367-2630/aaf544
Directing Bacterial Motion by Structured Surfaces and Wall Slip
The study and detailed understanding of the behaviour of biological microswimmers, such as sperm, bacteria, and algae, is of interest to establish better control and manipulaton of their motion, but also of the construction and optimization of artificial microswimmers for a variety of medical and technical tasks.
Peritrichous bacteria, such as escherichia coli and salmonella, are propelled by a bundle of rotating helical flagella. Hydrodynamic interactions imply circular trajectories near surfaces.
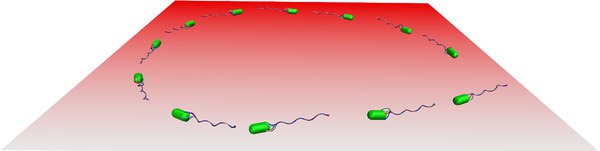
We have constructed a detailed mechanical model of such a bacterium. Its motion in a fluid near a wall is studied by mesoscale hydrodynamics simulations.The curvature and orientation (clockwise, counterclockwise) of the trajectory depend on the fluid boundary conditions at the surface. A quantitative study reveals its quantitative dependence on the boundary slip length. These results are then employed to propose a novel approach to directing bacterial motion on striped surfaces with different slip lengths, which implies a transformation of the circular motion into a snaking motion along the stripe boundaries.
The feasibility is demonstrated by a simulation of active Brownian rods, which also reveals a dependence of directional motion on the stripe width. This approach can be used for separating bacteria with different trajectory radii.
Swirls and Vortices in Suspensions of Self-Propelled Brownian Spheres
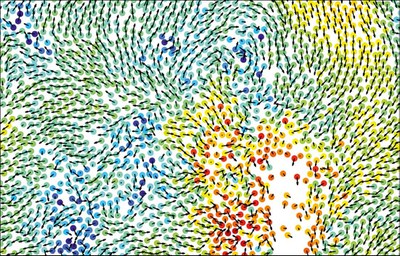
Assemblies of intrinsically active objects represent an exceptional class of non-equilibrium systems. Examples range from the macroscopic scale of human crowds to the microscopic scale of cells and motile microorganisms. A generic phenomenon in such systems is the emergence of self-organized large-scale dynamical patterns like vortices, swarms, networks, or self-sustained turbulence. This intriguing dynamics is a consequence of the complex interplay of self-propulsion, internal or external noise, and many-body interactions.
The understanding of collective behaviour requires the characterization of the underlying physical interaction mechanisms. Alignment induced by particle interactions, e.g., collisions between elongated objects or hydrodynamic interactions, is known to lead to clustering and collective motion. In contrast, we study suspensions of self-propelled Brownian spheres in three spatial dimensions, which lack any alignment mechanism.
Our simulations reveal a phase separation into a dilute and a dense phase above a certain density and strength of self-propulsion. The packing fraction of the dense phase approaches random close packing at high activity, yet the system remains fluid. Although no alignment mechanism exists, we find long-lived cooperative motion of particles in the dense regime, which is due to an interface-induced sorting process.
Bacterial Flagella: Synchronization, Bundling, Phase-Locking, and Slippage
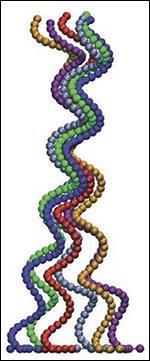
Peritrichous bacteria, such as E. coli, are covered by multiple flagella, which are essential for their locomotion. An individual flagellum is of helical shape and driven by a rotary motor attached to the bacterial cell body. When all left-handed flagella turn counterclockwise, they form a single helical bundle and the bacterium moves forward. This concerted motion of the bundle requires a synchronized rotation of the various flagella. The steady forward motion is interrupted by short periods of tumbling. This allows the bacteria to change their direction of motion, and to perform a biased random walk to adjust to environmental conditions, such as a search for food.
We investigate the synchronization and bundling process of bacterial flagella by mesoscale hydrodynamic simulations. A flagellum is modelled as a helical structure with bending and torsional rigidity. The characteristic times for synchronization and bundling are analyzed in terms of (equal) motor torques, separation, and number of flagella. Hydrodynamic interactions determine the bundling behaviour. The synchronization time is smaller than the bundling time, but their ratio depends strongly on the initial flagellar separation. Unbundling and tumbling is induced by a reduction of the torque of one flagellum. Small torque differences do not affect bundle stability due to phase locking, while large torque differences imply slippage and unbundling.
Emergence of Metachronal Waves in Cilia Arrays
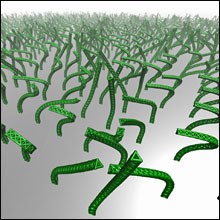
Propulsion by cilia is a fascinating and universal mechanism in biological organisms to generate fluid motion on the cellular level. Cilia are hair-like organelles, which are found in many different tissues and many uni- and multi-cellular organisms. Assembled in large fields, cilia beat neither randomly nor completely synchronously -- instead they display self-organization in the form of metachronal waves (MCWs). The main questions are how the individual cilia interact with the flow field generated by their neighbours and synchronize their beats for the metachronal wave to emerge, and how the properties of the metachronal wave are determined by the geometrical arrangement of the cilia, i.e. cilia spacing and beat direction.
We address these issues by using large-scale computer simulations of a mesoscopic model of two-dimensional cilia arrays in a three-dimensional fluid medium.We show that hydrodynamic interactions are indeed sufficient to explain the self-organization of MCWs, and study beat patterns, stability, energy expenditure and transport properties. We find that MCW strongly increase both propulsion velocity and efficiency -- compared to cilia all beating in phase. This can be a vital advantage for ciliated organisms, and may be interesting to guide biological experiments as well as the design of efficient microfluidic devices and artificial microswimmers.
Sperm Hydrodynamics near Surfaces
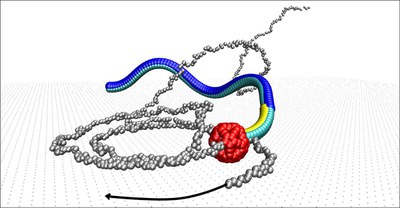
The sperm cell is probably the best-known example of a microscopic swimmer. Motion is generated by a snake-like motion of its tail, called the flagellum, which pushes the head (containing the genetic material) forward. This motion is essential for the sperm to reach the egg. Sperm fall into the general class of microswimmers called "pushers", because the forward force is generated in the rear part of the swimmer.
In confinement, sperm are found to accumulate at the walls and to swim in circles. Mesoscale hydrodynamics simulations can unravel the physical mechanism of this behavior. The reason for the attraction to the wall is the hydrodynamic flow field generated by the beating tail. The circling motion can be traced back to the chiral shape of sperm.
Hydrodynamic Interactions of Squirmers

Squirmers are microswimmers, for which a steady tangential surface velocity is prescribed on the surface of a spherical particle. This is a model for the flow generated by the time-averaged beating of cilia on a mesoscopic length scale, for example in volvox algae or paramecium. Squirmers may also serve as a simple generic model for other types of microswimmers, such as diffusiophoretic particles.
The motion and interactions of pairs of squirmers that are of micrometer size and therefore small enough to be subject to thermal noise, are governed by hydrodynamic forces and rotational diffusion. We demonstrate that hydrodynamic attraction dominates for squirmers moving initially in parallel, but misalignment of swimming directions due to thermal noise governs the motion after collision and implies diverging trajectories.
Self-Propelled Nanorods near Surfaces
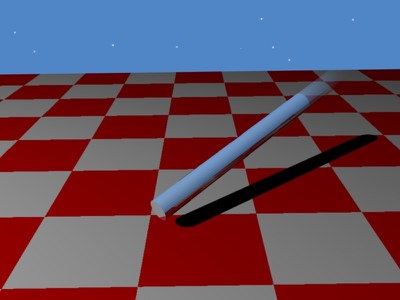
Self-propelled rods can also be seen as a generic model for a large class of self-propelled elongated particles, in case the details of the propulsion mechanism are not important. Passive elongated particles are repelled from a planar wall due to reduced entropy. Does this also apply to rod-like swimmers?
Computer simulations show that self-propelled rods actually align with and accumulate at the wall. This effect increases with increasing swimming velocity and with increasing rod length. It can be traced back to the difficulty of an aligned particle to wiggle itself free from the wall. There is an interesting analogy of the swimming trajectories with the conformations of semi-flexible polymers.
Cooperation of sperm in two dimensions
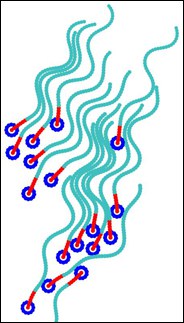
Sperm motility is important for the reproduction of animals. Sperm cells are propelled in a fluid by a snake-like beating of their tails. The sperm motion occurs in the regime of low Reynolds numbers, where inertia effects are negligible. The beating tails not only propel the sperm through the fluid, but also create flow fields through which sperm interact with each other. We study the hydrodynamic interaction and cooperation of sperm embedded in a two-dimensional fluid by using multi-particle collision dynamics (MPC), a particle-based mesoscopic simulation technique. Two effects of hydrodynamic interaction are found, synchronization and attraction. With these hydrodynamic effects, cooperating sperm clusters are formed in system at high concentrations. A dynamic balance between cluster formation and break-up results in a stable cluster-size distribution, which depends on the number density of sperm and on the distribution of beating frequencies of the individual sperm. The average cluster size shows a power-law dependence on the width of the frequency distribution.