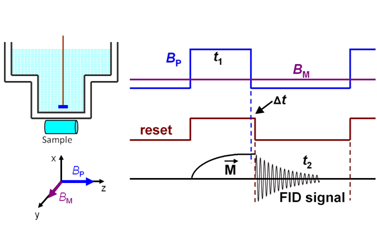
We perform nuclear magnetic resonance experiments at extremely low magnetic fields, using our nitrogen-cooled superconducting quantum interference device (SQUID) as a detector. A pulsed magnetic field Bp of a few Millitesla is used to polarize the sample. After switching off this polarization field, the free induction decay is observed in the detection field BM which is of the order of the earth’s magnetic field.
Compared to conventional nuclear magnetic resonance (NMR) at fields of a few tesla flux density, low field (LF) NMR at a few microtesla or less requires much less experimental effort and allows to study different relaxation processes. For a fixed relative homogeneity, the NMR line width scales linearly with the measurement field and makes the NMR lines very narrow at low fields, coming close to the lifetime-limited natural widths.
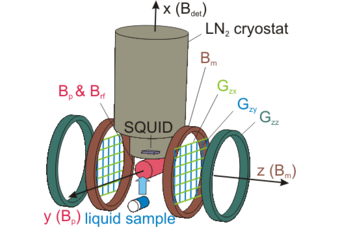
Our setup is schematically shown in the figure. The liquid sample is inserted into the solenoid which creates the polarizing field Bp. It is located beneath the fiberglass cryostat incorporating the SQUID sensor. The coil for the tipping field Bac is wound outside the Bp solenoid. A Helmholtz coil pair generates the measurement field Bm which is applied perpendicular to Bp. The gradient field Gzz is produced by a Maxwell coil pair. The other two gradient field components, Gzx and Gzy, are generated by pairs of planar grid coils, respectively.
LF-MRI measurements were performed with a piece of pepper. The size of the sample was about 22 mm in length and 17 mm in width. The transverse relaxation time T2* of the sample is estimated to be about 0.6 s and the amplitude of its free induction decay signal is approximately equivalent to that of 0.5 ml tap water. The reconstructed 2-D MRI images for different numbers of averages using the spin echo signal are shown in the figure. The geometrical shape of the sample is already reconstructed well even without averaging.
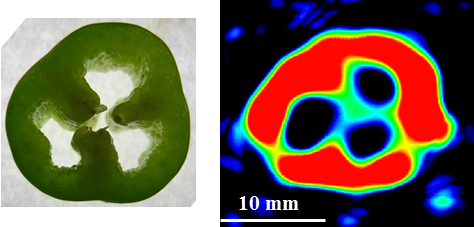
More Information
PUBLICATIONS:
Y. Li, P. Ma Q. Tao, H.-J. Krause, S. Yan, G. Ding, H. Dongr, X. Xie, Magnetic Graphene Quantum Dots Facilitate Closed-Tube One-Step Detection of SARS-CoV-2 with Ultra-Low Field NMR Relaxometry, Sensors and Actuators B 337, 129786 (2021).
X. Huang, H. Dong, Q. Tao, M. Yu, Y. Li, L. Rong, H.-J. Krause, A. Offenhäusser, X. Xie, Sensor Configuration and Algorithms for Power-line Interference Suppression in Low Field Nuclear Magnetic Resonance, Sensors 19, 3566 (2019).
X. Huang, H. Dong, Y. Qiu, B. Li, Q. Tao, Y. Zhang, H.-J. Krause, A. Offenhäusser, X. Xie, Adaptive suppression of power line interference in ultra-low field magnetic resonance imaging in an unshielded environment, J. Magn. Reson. 286, 52-59 (2018).
C. Liu, B. Chang, L. Qiu, H. Dong, Y. Qiu, Y. Zhang, H.-J. Krause, A. Offenhäusser, X. Xie, Effect of magnetic field fluctuation on ultralow field MRI measurements in the unshielded laboratory environment, J. Magn. Reson. 257, 8-14 (2015).
C. Liu, Y. Zhang, H. Dong, L. Qiu, H.-J. Krause, X. Xie, A. Offenhäusser, Tuned HTS SQUID-Detected Low Field MRI Using a Permanent Magnet for Pre-polarization With Automatic Transportation, IEEE Trans. Appl. Supercond. 23, 1601104 (2013).